Genetic Diseases
Understanding Genetic Diseases and the Promise of CRISPR Technology
Genetic diseases are disorders caused by abnormalities in an individual’s DNA. These abnormalities can be inherited from one or both parents or can occur spontaneously due to mutations. They often manifest as a variety of health issues, ranging from relatively benign conditions to severe, life-threatening disorders. Examples of genetic diseases include cystic fibrosis, sickle cell anemia, Huntington's disease, and muscular dystrophy. Traditional approaches to treating genetic diseases have typically focused on managing symptoms rather than addressing the underlying genetic causes. However, advances in gene-editing technologies, particularly CRISPR (Clustered Regularly Interspaced Short Palindromic Repeats), are revolutionizing the way researchers approach genetic diseases.
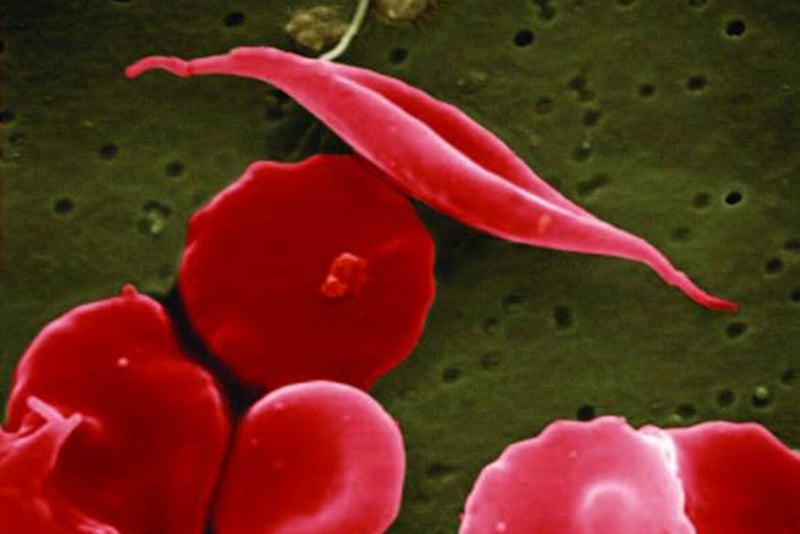
Figure 1. Sickle cell disease. (Credit: Boston University)
The CRISPR Revolution
CRISPR is a groundbreaking gene-editing technology that allows scientists to make precise, targeted changes to an organism's DNA. It works by utilizing a bacterial immune system component, known as CRISPR-Cas9, which can be programmed to target specific sequences of DNA. Once the target is located, the Cas9 enzyme cuts the DNA at the desired location, allowing for the removal, addition, or alteration of specific genetic material.
This technology has rapidly gained prominence since its development in the early 2010s due to its versatility, precision, and relatively low cost compared to earlier gene-editing methods. CRISPR has opened up new possibilities for treating genetic diseases by enabling the correction of defective genes at their source, potentially providing cures rather than merely palliative care.
CRISPR and Genetic Disease Research
One of the most significant applications of CRISPR in the context of genetic diseases is its use in research. Scientists are using CRISPR to create disease models, which are essential for understanding the underlying mechanisms of genetic disorders and for developing new treatments.
In traditional research, creating animal models of genetic diseases often involved laborious and time-consuming breeding techniques. However, CRISPR allows researchers to introduce specific mutations into the genomes of animals like mice, zebrafish, or even non-human primates, which can then be studied to understand how these mutations lead to disease. These models provide invaluable insights into the pathophysiology of genetic disorders and can be used to test potential treatments before they are used in humans.
Moreover, CRISPR is also being used to engineer human cells that carry specific genetic mutations. By editing the genomes of cultured cells, researchers can create cellular models of diseases, such as heart cells with mutations associated with cardiomyopathy or neurons with mutations linked to neurodegenerative diseases. These models are particularly useful for studying diseases that are difficult to model in animals and for screening drugs that might reverse or mitigate the effects of genetic mutations.
CRISPR in Clinical Applications: Gene Therapy and Beyond
While CRISPR is transforming research, its potential in clinical applications is equally revolutionary. Gene therapy, which aims to treat or prevent disease by directly altering the patient’s genetic material, is one of the most promising areas where CRISPR could make a significant impact.
Several clinical trials are already underway to test CRISPR-based therapies for genetic diseases. For example, CRISPR has been used in trials to treat sickle cell anemia and beta-thalassemia, both of which are blood disorders caused by mutations in the hemoglobin gene. In these trials, researchers extract hematopoietic stem cells (which give rise to blood cells) from patients, use CRISPR to correct the defective gene in these cells, and then reintroduce the edited cells back into the patient. Early results from these trials have been promising, with some patients showing significant improvements in their condition.
Another area of active research is using CRISPR to treat inherited blindness. In 2020, a CRISPR-based therapy was administered directly to the retina of a patient with Leber congenital amaurosis, a genetic disorder that leads to blindness. This marked the first time CRISPR was used in vivo (directly inside a patient’s body), representing a significant milestone in the development of gene therapies.
However, while the potential of CRISPR-based therapies is immense, there are also significant challenges that need to be addressed. One of the primary concerns is the possibility of off-target effects, where the CRISPR system inadvertently edits DNA at unintended locations, which could lead to new mutations or other unintended consequences. As a result, much research is focused on improving the precision and safety of CRISPR-based therapies.
Another challenge is the delivery of CRISPR components to the correct cells within the body. Currently, most gene-editing therapies rely on viral vectors to deliver CRISPR-Cas9 to target cells, but this method has limitations, including immune responses and difficulties in targeting specific tissues. Researchers are exploring alternative delivery methods, such as lipid nanoparticles, to overcome these challenges.
.png?width=1470&name=unnamed%20(94).png)
Figure 2. iPSC-based disease modeling for human diseases.
Transforming Genetic Disease Research with EditCo's CRISPR Innovation
Intelligent Guide Design
EditCo’s innovative approach to smart guide design allows efficient gene disruption generation, eliminating the trial and error associated with common guide design strategies. All our reagents are designed to streamline your research, ensuring more accurate and reliable results.
Target Identification & Validation: Finding & Confirming Therapeutic Targets with Confidence
With intelligent guide design strategy, EditCo is able to simply and effectively knockout genes guaranteed with our Gene Knockout Kits or do high-throughput genomic screening with Arrayed gRNA Libraries, allowing for clear genotype-to-phenotype answers for many genes at scale and quickly.
Using CRISPR to knockout genes at various scales allows researchers to quickly identify potential oncogenic drivers, gene-gene interactions, or drug resistance pathways. The arrayed screening libraries, such as EditCo’s Arrayed gRNA Libraries or unique Engineered Cell Libraries, allow for one gene to be targeted per well across multiwell plates, creating straightforward genotype-phenotype connections with minimal data analysis. Additionally, pre-designed screening libraries can also include cancer-specific gene families like the complete druggable genome, G protein-coupled receptors (GPCRs), and immunology/immuno-oncology genes), custom gene libraries, or even the whole human/mouse genome.
Target validation is the confirmation of identified targets from target screening workflows. Secondary confirmation of genotype-phenotype interactions is critical to ensure confidence in proceeding toward complex functional assays with novel drug targets. Confidence in a functional knockout for a particular gene is necessary to study cancer-specific processes such as the epithelial-mesenchymal transition, where assay sensitivity is critical. Improving the reliability of this step, with either guaranteed CRISPR knockout reagents, like Gene Knockout Kits, or simply using custom-edited Immortalized Knockout Cells, can make it easier.
Lead Identification and Optimization: Evaluate Therapies, Not Cell Models
Lead validation confirms candidate new molecular entities, biologics, or cell gene therapies for a particular disease indication. In cancer contexts, this step evaluates these therapeutics against model cell lines carrying relevant oncogenic or drug resistant genotypes. The data generated from these experiments is critical for the continued optimization of lead therapeutics. Moreover, certainty in the genotype of challenged cell models or modified cell gene therapies is integral in highly sensitive assays such as transcriptomics. Cell models with a complete phenotypic knockout and defined genetic background, such as EditCo’s Knockout and Knock-in Immortalized Cell Clones, are critical for ensuring sensitivity in evaluating lead therapeutic indications in complex downstream phenotypic assays.
Have more questions? Reach out to us!